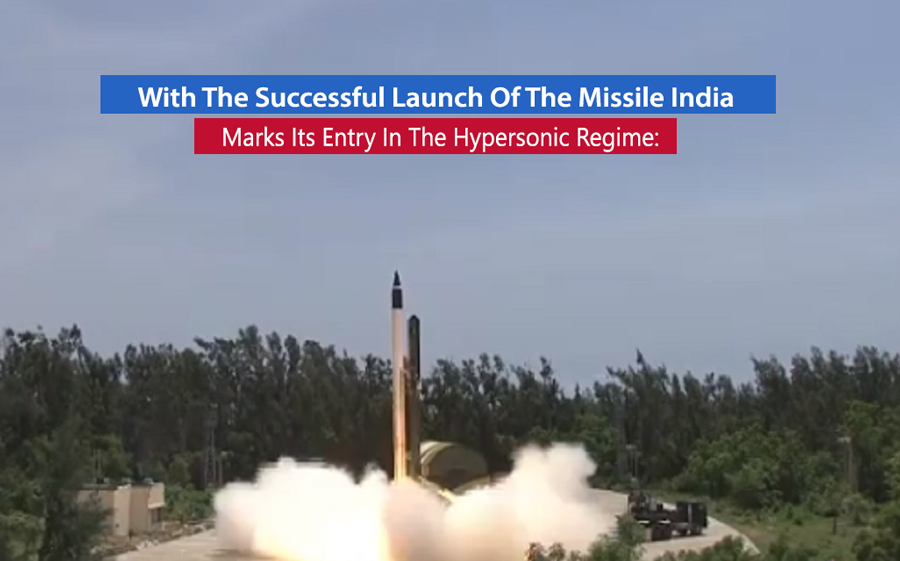
On September 2020, Defence Research and Development Organization of India has demonstrated successfully the Hypersonic Air-Breathing Scramjet technology with the flight test of (HSTDV) Hypersonic Technology Demonstration Vehicle at 1103 hours from Dr APJ Abdul Kalam Launch Complex at Wheeler Island, off the coast of Odhisha.
The Hypersonic cruise vehicle was launched by using a proven solid rocket motor that took it on an altitude of 30 km, where the aerodynamic heat shields were separated at the hypersonic Mac number. This cruise vehicle separated from the launch vehicle continued on its desired flight path at a velocity of six times the speed of sound that is nearly 2km/s for more than 20seconds.
For aircraft speeds that are much greater than the sound speed, the aircraft is said to be hypersonic. Typical speeds for hypersonic aircraft are excellent and greater than 3000 mph and Mach number M greater than five, M > 5. The main characteristic of hypersonic aerodynamics is the temperature of the flow is so great that the chemistry of the diatomic molecules of the air must be considered. At low hypersonic speeds, the molecular bonds vibrate, that modifies the magnitude of the forces created by the air or the aircraft. At the high hypersonic speeds, the molecules break apart producing the electrically charged plasma across the aircraft. Large variations in air density and pressure happen due to the shock waves and expansions.
Supersonic airflow is decidedly several from the subsonic flow. Nearly everything about the way an aircraft file changes radically as an aircraft accelerates to supersonic speeds. Even with this strong demarcation, there is still some debate as to the definition of “supersonic”. One definition is that the aircraft, as a whole, is travelling at Mach 1 or greater. More technical definitions state that supersonic speed is achieved only if the airflow over the whole aircraft is supersonic that happens across Mach 1.2 on typical designs. The range Mach 0.75 to 1.2 is hence considered transonic.
Flow Characteristics
While the definition of hypersonic flow can be quite vague and is commonly debatable, a hypersonic flow might be characterized by certain physical phenomena that can no longer be analytically discounted as in supersonic flow. These phenomena include:
- Thin Shock Layer
As Mach numbers increase, the density behind the shock also increases, that corresponds to a decrease in the volume behind the shock wave because of the conservation of mass. Therefore, the shock layer, that volume between the body and the shock wave is thin at high Mach numbers.
- Entropy Layer
As Mach numbers increase, the entropy change around the shock also increases that in results a string entropy gradient and highly vertical flow that mixes with the boundary layer.
- Viscous Interaction
A portion of the large kinetic energy linked with the flow at high Mach numbers adapts into internal energy in the fluid because of viscous effects. The increase is internal energy is realized as an increase in temperature. Since the pressure gradient normal to the flow within a boundary layer is zero, the increase of temperature via the boundary layer coincides with the decrease in density. Therefore, the boundary layer over the body grows and can often merge with the think shock layer.
- High-Temperature Flow
High temperature discussed previously as a manifestation of viscous dissipation cause non-equilibrium chemical flow properties like dissociation and ionization of molecules resulting in convective and radiative heating.
Regimes
Hypersonic flow can be separated approximately into a number of regimes. The selection of these regimes is rough, because of the blurring of the boundaries where a particular effect can be found.
Perfect Gas
In this regime, the gas can be regarded as an ideal gas. Flow in this regime is still Mach number dependent. Simulations start to depend on the use of a constant-temperature wall, rather than the adiabatic wall commonly used at lower speeds. The lower border of this region is across Mach 5, where Ramjets become inefficient, and the upper border across Mach 10-12.
Two-Temperature Gas
This is a subset of the perfect gas regime, where the gas can be measured chemically perfect but the vibrational and rotational temperatures of the have must be considered separately, leading to two temperature models. Modelling of supersonic nozzles, where vibrational freezing becomes essential.
Dissociated Gas
In this regime, multimolecular gases start to dissociate as they come into contact with the bow shock created by the body. The type of has chosen starts to have an effect on the flow and surface catalycity plays a role in the calculation of surface heating that means that the selection of the surface material also starts to have an effect on the flow. The lower border of the regime is where the first and foremost component of a gas combination starts to dissociate in the stagnation point of flow and the lower border of this regime is where the first component of a gas mixture starts to dissociate in the stagnation point of flow. The upper border of this regime is where the effects of ionization start to have an effect on the flow.
Ionized Gas
In this regime the ionized electron population of the stagnated flow becomes essential, and the electrons should be modelled separately. Often the electron temperature is handled separately from the temperature of the remaining gas components. The region happens for freestream velocities across 10-12 km/s. Gases in this region are modelled as non-radiating plasmas.
Radiation-Dominated Regime
Above across 12km/s, the heat transfer to a vehicle changes from being conductively dominated to radiatively dominated. The modelling of gases in this regime in this regime is split into two classes:
- Optically thin: Where the gas doesn’t re-absorb radiation emitted from other parts of the gas
- Optically thick: Where the radiation should be considered as a separate source of energy
The modelling of optically thick gases is extremely difficult, since, because of the calculation of the radiation at every point, the computation load theoretically expands exponentially as the number of points considered increases.